Dark matter influences cosmic behavior through gravitational interactions, leading to phenomena like “dark stars,” which may explode similarly to supernovae. The exploration of potential dark matter particles, such as WIMPs and axions, is critical to understanding these celestial events. Credit: SciTechDaily.com
Dark matter, influencing the universe through gravitational interactions, remains elusive with potential forms like WIMPs and axions, the latter possibly forming explosive ‘dark stars.’
Dark matter is a ghostly substance that astronomers have failed to detect for decades, yet which we know has an enormous influence on normal matter in the universe, such as stars and galaxies. Through the massive gravitational pull it exerts on galaxies, it spins them up, gives them an extra push along their orbits, or even rips them apart.
Like a cosmic carnival mirror, it also bends the light from distant objects to create distorted or multiple images, a process which is called gravitational lensing.
And recent research suggests it may create even more drama than this, by producing stars that explode.
The Quest to Identify Dark Matter
For all the havoc it plays with galaxies, not much is known about whether dark matter can interact with itself, other than through gravity. If it experiences other forces, they must be very weak, otherwise, they would have been measured.
A possible candidate for a dark matter particle, made up of a hypothetical class of weakly interacting massive particles (or WIMPs), has been studied intensely, so far with no observational evidence.
Recently, other types of particles, also weakly interacting but extremely light, have become the focus of attention. These particles, called axions, were first proposed in late 1970s to solve a quantum problem, but they may also fit the bill for dark matter.
Axions and the Cosmic Dance
Unlike WIMPs, which cannot “stick” together to form small objects, axions can do so. Because they are so light, a huge number of axions would have to account for all the dark matter, which means they would have to be crammed together. But because they are a type of subatomic particle known as a boson, they don’t mind.
In fact, calculations show axions could be packed so closely that they start behaving strangely – collectively acting like a wave – according to the rules of quantum mechanics, the theory which governs the microworld of atoms and particles. This state is called a Bose-Einstein condensate, and it may, unexpectedly, allow axions to form “stars” of their own.
This would happen when the wave moves on its own, forming what physicists call a “soliton,” which is a localized lump of energy that can move without being distorted or dispersed. This is often seen on Earth in vortexes and whirlpools, or the bubble rings that dolphins enjoy underwater.
The new study provides calculations that show that such solitons would end up growing in size, becoming a star, similar in size to, or larger than, a normal star. But finally, they become unstable and explode.
The energy released from one such explosion (dubbed a “bosenova”) would rival that of a supernova (an exploding normal star). Given that dark matter far outweighs the visible matter in the universe, this would surely leave a sign in our observations of the sky. We have yet to find such scars, but the new study gives us something to look for.
Observational Prospects and Theoretical Developments
The researchers behind the study say that the surrounding gas, made of normal matter, would absorb this extra energy from the explosion and emit some of it back. Since most of this gas is made of hydrogen, we know this light should be in radio frequencies.
Excitingly, future observations with the Square Kilometre Array radio telescope may be able to pick it up.
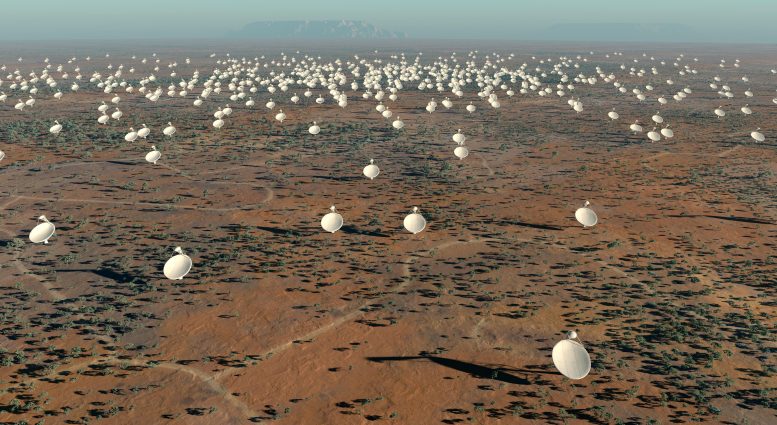
This artist’s impression shows the Square Kilometer Array, a telescope array currently being built in both Australia and Africa. Credit: SPDO/TDP/DRAO/Swinburne Astronomy Productions
So, while the fireworks from dark star explosions may be hidden from our view, we might be able to find their aftermath in the visible matter. What’s great about this is that such a discovery would help us work out what dark matter is actually made of – in this case, most likely axions.
What if observations will not detect the predicted signal? That probably won’t rule out this theory completely, as other “axion-like” particles are still possible. A failure of detection may indicate, however, that the masses of these particles are very different, or that they do not couple with radiation as strongly as we thought.
In fact, this has happened before. Originally, it was thought that axions would couple so strongly that they would be able to cool the gas inside stars. But since models of star cooling showed stars were just fine without this mechanism, the axion coupling strength had to be lower than originally assumed.
Of course, there is no guarantee that dark matter is made of axions. WIMPs are still contenders in this race, and there are others too.
Incidentally, some studies suggest that WIMP-like dark matter may also form “dark stars”. In this case, the stars would still be normal (made of hydrogen and helium), with dark matter just powering them.
These WIMP-powered dark stars are predicted to be supermassive and to live only for a short time in the early universe. But they could be observed by the James Webb space telescope. A recent study has claimed three such discoveries, although the jury is still out on whether that’s really the case.
Nevertheless, the excitement about axions is growing, and there are many plans to detect them. For example, axions are expected to convert into photons when they pass through a magnetic field, so observations of photons with a certain energy are targeting stars with magnetic fields, such as neutron stars, or even the Sun.
On the theoretical front, there are efforts to refine the predictions for what the universe would look like with different types of dark matter. For example, axions may be distinguished from WIMPs by the way they bend the light through gravitational lensing.
With better observations and theory, we are hoping that the mystery of dark matter will soon be unlocked.
Written by Andreea Font, Reader in Theoretical Astrophysics, Liverpool John Moores University.
Adapted from an article originally published in The Conversation.